Trans-Neptunian objects (TNOs) are icy bodies ranging in size from Pluto and Eris (dwarf planets with diameters of about 1,500 miles) down to tens of miles (Arrokoth) and even smaller. TNOs are on orbits comparable in size, or even much larger than, that of Neptune. The existence of TNOs was postulated by Kenneth Edgeworth, and later by Gerard Kuiper, in the 1950s; the region of space occupied by TNOs is usually referred to as the Kuiper Belt, and TNOs themselves, sometimes referred to as Kuiper Belt objects (KBOs).
The orbits of TNOs are extremely diverse but fall into groupings that reflect the outward migration of Uranus and Neptune early in the history of the formation of the solar system. As such, TNOs hold the keys to understanding that early history. However, it took NASA’s James Webb Space Telescope and its unparalleled ability to study the materials on the surfaces of TNOs to fully begin to grasp what they can tell us about our origins. Here Bryan Holler and John Stansberry from the Space Telescope Science Institute (STScI) in Baltimore describe how Webb is expanding our knowledge of these objects.
Of the TNOs whose orbits were perturbed during giant-planet migration, it is difficult to trace them back to where they formed. Yet it is only by studying the composition of individual TNOs that we can hope to map out the composition of the primordial outer disk. TNOs are on very distant orbits from the Sun, and are very cold, below minus 280 degrees Fahrenheit (about minus 170 degrees Celsius), so their surfaces could provide information about the original composition of planetesimals within the disk. Webb is the first observatory able to provide detailed compositional information about typical TNOs (with diameters less than about 500 miles, or 800 kilometers) because of its large primary mirror and highly sensitive instruments. In particular, the Near Infrared Spectrograph (NIRSpec) has for the first time revealed TNO compositions in exquisite detail.
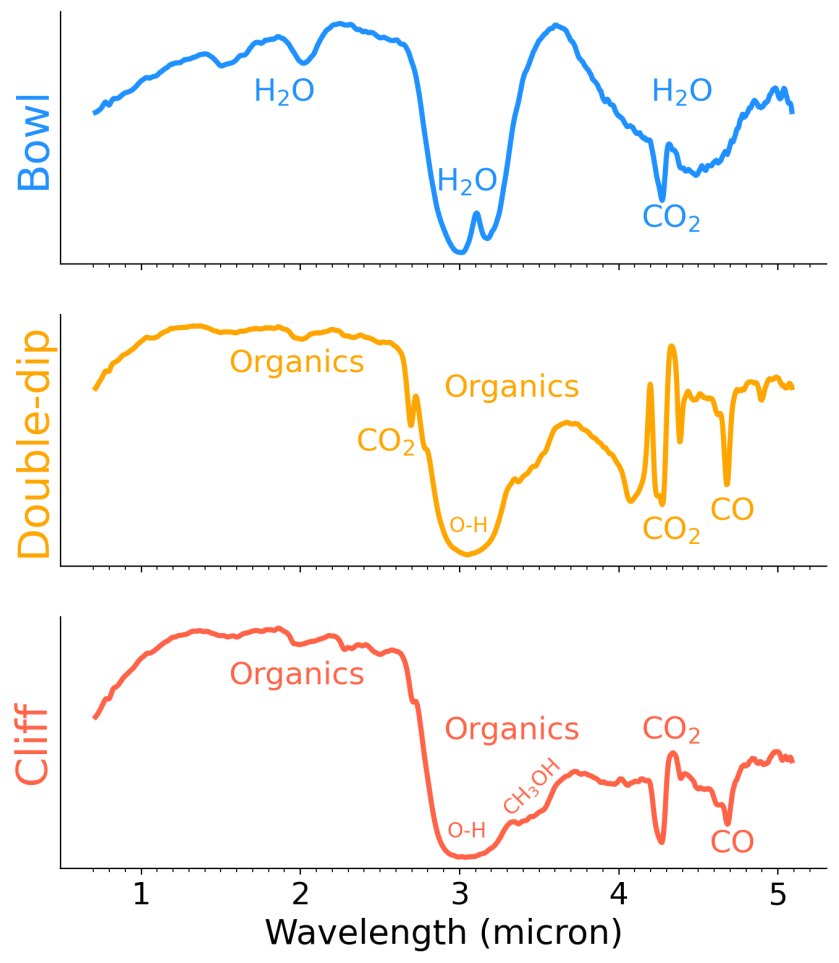
Webb’s NIRSpec divides light at wavelengths between about 1 and 5 microns into hundreds or thousands of individual colors. The relative brightness of those colors as a function of wavelength is a spectrum. Different materials exhibit different spectra that help identify the composition of the object observed. Because the TNOs formed in the cold, outer portions of the protoplanetary disk, it has long been expected that they would have surfaces dominated by ices of molecules that are gases or liquids at Earth’s surface, e.g. water (H2O), carbon dioxide (CO2), nitrogen (N2), and methane (CH4), among others. Further, radiation from the Sun and outside the solar system alters the chemistry, creating new, more complex hydrocarbon (organic) molecules such as methanol (CH3OH), acetylene (C2H2), and ethane (C2H6). Webb data has confirmed this, but in unexpected ways, and in unprecedented detail.
Within the first two years of science operations, Webb has taken high-quality spectra of over 75 TNOs and provided the first comprehensive look at what they are made of, including nearly 60 objects from the Large Cycle 1 program called “DiSCo-TNOs” (program ID #2418, PI: Noemí Pinilla-Alonso). The major result from the large dataset from the DiSCo-TNOs program is the identification of three spectral classes, which is the first evidence for distinct surface compositions, that was completely unexpected based on earlier studies. These classifications are named based on the spectral shape in the 2.5–4 micron region, with the deepest band centered at 3.0 microns generated by molecules that contain an oxygen-hydrogen bond, such as water. Bowl-type spectra are dominated by the absorption features of water ice, with some carbon dioxide ice, and indications of silicate-rich dust. Double-dip spectra have absorption features due to complex organic molecules, carbon dioxide, and carbon monoxide ices. Cliff spectra have even more complex organic materials and carbon dioxide than Double-dips, and also include features due to CH3OH. Double-dip spectra indicate very abundant and pure carbon dioxide ice, as evidenced by the two reflectance peaks (never observed outside of a laboratory) bounding the 4.27 micron band. The three spectral types are also distinct in their color at the shortest visible wavelengths, with bowls being least red, double-dips intermediate, and cliffs reddest.
The DiSCo-TNOs team hypothesizes in Pinilla-Alonso et al. (2024) that these different spectral types are the result of higher temperatures closer to the Sun, and colder temperatures farther out. Specifically, the Bowl types formed closer to the Sun and were subject to higher temperatures that essentially baked off the carbon dioxide and methane. These compounds were more stable on Double-dips and Cliffs, which formed farther out. An important clue leading to that hypothesis is that all of the objects on undisturbed cold-classical orbits are Cliffs. TNOs on other orbits include objects from all three compositional types, as would be expected due to the dynamical reshuffling as Neptune migrated outward, as described above.
Looking ahead, Webb continues to carry out a robust program of TNO observations each year, with new and exciting programs selected by the community for execution. Cycle 3 will see imaging and spectroscopy of a handful of TNOs and their satellites, including the first-ever spectral observations of the “extreme” TNOs, with orbits that take them well into interstellar space. Another program aims to circle back to targets observed in the first year of science operations to get an even more detailed look at the materials that led to the formation of TNOs in the protosolar nebula. Two other programs focus on imaging and spectroscopy of TNO binary systems to better understand the origins of TNO satellites, either via giant impacts or co-formation via gravitational collapse. Who knows what new ideas and exciting discoveries the next year will bring?
Editor’s Note: These findings have been published in Nature Astronomy.
Related Links:
The full list of team members for General Observers program 2418.
About the Authors
John Stansberry, STScI observatory scientist and Webb solar system lead at STScI, helped define the science goals and implementation of the observations in program 2418 (DiSCo-TNOs) and interpreted the data as reflecting the formation distances of the individual TNOs in the proto-planetary disk.
Bryan Holler, STScI scientist and Webb solar system deputy lead at STScI, processed and extracted spectra from the NIRSpec data for the DiSCo-TNOs program.