This week, guest blogger Jared Austin, a fellow writer on the SLS Strategic Communications team, is back for a look at an example of the innovative technology work taking place in the SLS program. — David

Would you like a cellphone, tablet, or laptop that is lighter and more powerful and will recharge for use in a matter of seconds? If your answer is “Yes!” then the Space Launch System Program is working to make your life a little bit better. (If your answer is “no,” are you sure you’re in the right place?)
Rockets and smartphones may not seem like they have much in common, but one thing they do share is a need for reliable power for electronic systems. And that’s where ultracapacitors come in.
Ultracapacitors are small devices, as small as roughly the size of a business card, which means more room and less weight than a traditional battery. On top of that, ultracapacitors charge rapidly.
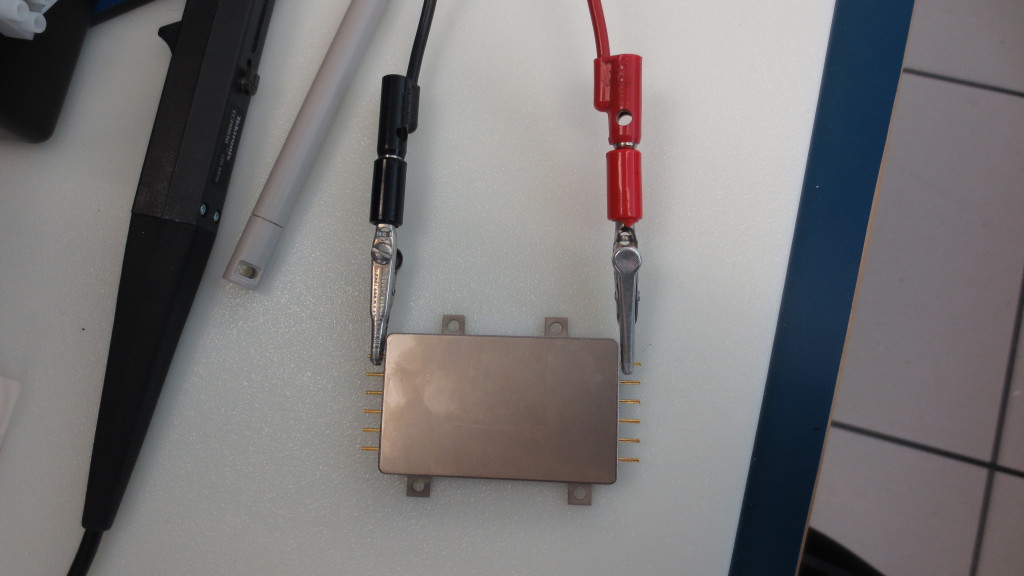
NASA’s new Space Launch System will be NASA’s exploration ride for decades to come, and that presents a unique challenge. While the first version of the rocket will be ready to fly in three years, NASA will continue to evolve it into more powerful configurations through the 2020s, meaning that NASA engineers are working today to make sure the final version of the rocket will still be state-of-the-art when it flies. To accomplish that, engineers are already engaged in the long-lead work of maturing new technologies for spaceflight.
For instance, every NASA system – from small “CubeSats” to rovers, satellites, and even rockets – has an electrical system that requires power. The most common power source is a battery. Despite their widespread use, rechargeable batteries come with a number of drawbacks. They are slow to charge up (hours) and are necessarily bulky in order to meet power requirements. Batteries heat up during use (feel the bottom of your laptop after an hour’s use), and that overheating can wear on the device its powering. On top of that, batteries contain harmful chemicals that are bad for the environment, and suffer from early wear-out, especially in the harsh environments of space where many NASA systems operate.
Meet avionics failure analyst Terry Rolin, who kept seeing battery failures that were creating problems for NASA systems.
“I have learned that failures present opportunities for individuals to learn new ways of doing things, build character, and teach new pathways for problem solving,” Terry said.

In 2012, he learned about ultracapacitors and was sure he had found the future.
The ultracapacitors can be significantly smaller than batteries, allowing more room for payloads, which is especially important to CubeSats where every centimeter matters. Ultracapacitors do not heat up during use, which is good for the system it’s powering. Also, due to their solid-state design (batteries contain a liquid core), the harsh environments of space – radiation, extreme temperatures, and pressures – do not affect them the same way they do batteries.
While ultracapacitors pose substantial promise for spaceflight applications, there was one major issue that had to be resolved before they could be rocket-ready. To build the compact ultracapacitors in a way that would maximize their capacity to store energy, they need to be as thin as possible; an ultracapacitor’s ability to store electrical charges actually decreases as it grows in size. Terry and his team won an innovation fund in the summer of 2012 to develop ultracapacitors that could power NASA systems using 3-D printing techniques and nanotechnology in order to manufacture the smaller ultracapacitors.
For SLS, despite the enormous size of the rocket, there are numerous small avionics computer boxes requiring power systems that fit in a tight space. Ultracapacitors are being evaluated for use down the road, either as the primary power system, or in a hybrid combination with batteries taking advantage of the best of both power sources.
“I was seeing increases in the ability of the capacitors to store energy by several orders of magnitude, and getting charging times of seconds rather than hours,” Terry said. “It was very exciting.”
But Terry’s team had difficulty reproducing their efforts. They found micro- and nano-fractures in their ultracapacitors, so they reached out to industry and academia for help.

It turned out that the nanoparticles in the commercial electrode ink they were using during the 3-D printing process the devices required a temperature so high that it damaged the ultracapacitors. So, Terry’s team developed a new ink that would sinter, or “print,” at lower temperatures, but still work for commercial manufacturing processes. Because of this work, down the road, commercial companies could release a nanoparticle ink that lets anyone with a 3-D printer to create their own ultracapacitors to power their electronics.
While their full potential is still being researched by Terry and his team in the space systems department at NASA’s Marshall Space Flight Center, ultracapacitors show promise to not only make NASA systems smaller, lighter, more durable, easily rechargeable, and more environmentally friendly, but to potentially bring those same benefits to electronic devices in your home or pocket.
Ultracapacitors are only one of the many technologies the SLS program has been developing, both internally with NASA engineers and in partnership with industry and academia. NASA has a long history of “spin-offs” that have taken space system research to make lives on Earth better, from Hubble software that helps with cancer detection to filtration systems that help provide clean drinking water in remote areas.
A rocket for missions to Mars that makes life better on Earth? That truly is the best of both worlds.
Next Time: Hey, Want A Ride?
Join in the conversation: Visit our Facebook page to comment on the post about this blog. We’d love to hear your feedback!